Innovative miniature chips transform light into microwaves
This groundbreaking demonstration distinguishes itself through the compact design of the signal-producing components.
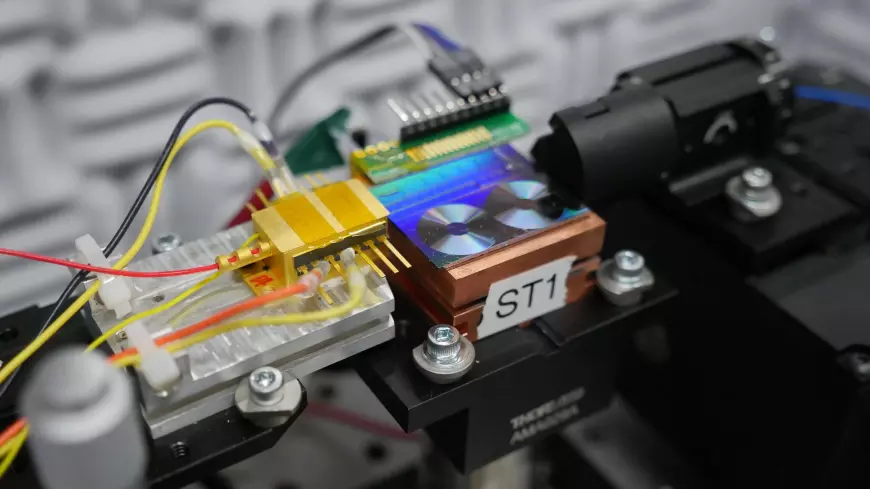
The National Institute of Standards and Technology (NIST) and its collaborators have achieved a significant breakthrough in timing technology, unveiling miniature chips that effortlessly convert light into microwaves. This chip holds the potential to enhance various technologies, including GPS, phone and internet connections, radar accuracy, and sensing systems, by providing high-precision timing and communication. The researchers have successfully reduced timing errors to an incredibly minute fraction of a second—precisely 15 femtoseconds, marking a substantial improvement over conventional microwave sources. This advancement ensures much greater stability and precision in signals, offering benefits such as heightened radar sensitivity, improved accuracy for analog-to-digital converters, and clearer astronomical images obtained through telescope arrays.
Shining a light on microwaves
This groundbreaking demonstration distinguishes itself through the compact design of the signal-producing components. In a notable achievement, researchers have successfully minimized a once-tabletop-size system into a compact chip, comparable in size to a digital camera memory card. The reduction in timing errors on this smaller scale not only lowers power consumption but also enhances the adaptability of the technology for everyday devices.
Presently, some components of this technology are external to the chip as researchers evaluate their effectiveness. The overarching objective is to integrate all the disparate elements—such as lasers, modulators, detectors, and optical amplifiers—onto a single chip. This integration would result in a significant reduction in both size and power consumption, facilitating seamless incorporation into compact devices without requiring extensive energy and specialized training.
Frank Quinlan, a physical scientist at NIST, highlighted the current complexities involved in generating microwave signals, requiring multiple laboratories and expertise. The focus of this research is leveraging the advantages of optical signals by miniaturizing components and enhancing accessibility.
To achieve this, researchers employ a semiconductor laser, functioning as a stable light source. The laser's light is directed into a small mirror box referred to as a reference cavity, akin to a miniature room where light undergoes reflection. Within this cavity, specific light frequencies align with the cavity's dimensions, leading to the perfect fit of light wave peaks and valleys between the walls.
This phenomenon results in the accumulation of power in those frequencies, maintaining the laser's frequency stability. The stable light is then transformed into microwaves through a device known as a frequency comb, converting high-frequency light into lower-pitched microwave signals. These precise microwaves play a crucial role in technologies like navigation systems, communication networks, and radar by providing accurate timing and synchronization.
Quinlan emphasized the project's two-phase approach: the first phase demonstrating the effective collaboration of individual components, and the second phase involving their integration onto the chip.
In navigation systems like GPS, precise signal timing is vital for location determination. Communication networks, including mobile phones and the internet, rely on accurate timing and synchronization to ensure proper data transmission and reception. For instance, in busy cell networks handling multiple phone calls, precise signal alignment enables efficient organization and management of data transmission without significant delays or drops.
In radar applications, used for detecting objects such as airplanes and weather patterns, precise timing is essential for accurately measuring signal travel times.
The technology's applications are diverse, spanning fields such as astronomy, where low-noise signals and clock synchronization are crucial for imaging distant objects like black holes. This project aims to transition these low-noise signals from the laboratory to the hands of professionals in various fields, enhancing sensitivity and measurement capabilities in areas like radar technology, astronomy, and environmental science.
Working together toward a shared goal
The development of this technological breakthrough was a collaborative effort, involving researchers from various institutions including the University of Colorado Boulder, the NASA Jet Propulsion Laboratory, the California Institute of Technology, the University of California Santa Barbara, the University of Virginia, and Yale University. Together, these researchers united in a shared objective: to transform the utilization of light and microwaves for practical applications.
Frank Quinlan likened the research process to a construction project, emphasizing the coordination required among the different contributors, similar to ensuring that plumbers and electricians arrive at the right time in a construction project. According to Quinlan, the success of the project hinges on the seamless collaboration of all involved parties, maintaining a collective focus on advancing the initiative.
This collaborative endeavor highlights the significance of interdisciplinary research in propelling technological advancements, as emphasized by Quinlan.