What is Quantum computing? Detailed explanation by Digimagg
Unveil the power of quantum computing: a revolutionary technology poised to transform computation and problem-solving.
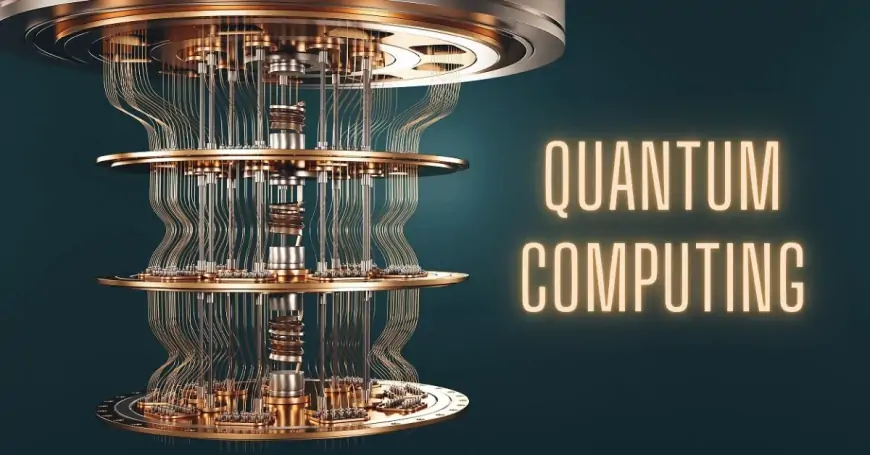
When scientists seek to leverage the capabilities of molecules, such as in photosynthesis, traditional computers fall short. They turn to quantum computers, adept at analyzing quantum systems at the molecular scale and calculating conditional probabilities. Essentially, quantum computers can execute computations equivalent to billions of years' worth in a mere weekend, unraveling some of the globe's most intricate challenges along the way.
What is Quantum computing?
Quantum computing leverages the principles of quantum mechanics to address problems of immense scale or complexity beyond the capabilities of conventional computers. These systems operate using qubits to execute and solve multidimensional quantum algorithms.
Quantum computing differs significantly from classical computing. Quantum physicist Shohini Ghose from Wilfrid Laurier University draws a comparison between the two by likening classical computing to candles and quantum computing to light bulbs, emphasizing that the latter is not just an improved version of the former but an entirely distinct entity.
Quantum computing
Quantum computing tackles mathematical challenges and executes quantum simulations based on the principles of quantum theory. It's employed to model various quantum systems like photosynthesis, superconductivity, and intricate molecular structures. To grasp quantum computing and its mechanisms, comprehension of concepts such as qubits, superposition, entanglement, and quantum interference is essential.
What are Qubits?
Qubits, the fundamental units of information in quantum computing, are analogous to traditional binary bits. However, unlike binary bits that can only represent 0 or 1, qubits leverage superposition to exist in multiple states simultaneously. This means they can embody both 0 and 1, as well as any combination of these states in superposition.
The composition of qubits varies depending on the architecture of quantum systems. Some systems necessitate extremely low temperatures for optimal functionality. Qubits can be constructed from a variety of materials, including trapped ions, photons, artificial or natural atoms, or quasiparticles. In contrast, binary bits are typically found in silicon-based chips.
What is Superposition?
When illustrating superposition, some individuals reference Schrödinger’s cat, while others use the analogy of a coin in mid-air during a toss. Essentially, quantum superposition denotes a state where quantum particles exist as a blend of all feasible states, continually fluctuating and evolving while the quantum computer observes and measures each particle.
John Donohue, scientific outreach manager at the University of Waterloo’s Institute for Quantum Computing, emphasizes that the intriguing aspect of superposition lies not just in the notion of simultaneous existence, but in the capacity to examine quantum states from multiple perspectives and pose diverse inquiries. Unlike traditional computers, which perform tasks sequentially, quantum computers can execute numerous parallel computations.
This explanation offers a simplified understanding before delving into equations. The primary takeaway is that superposition enables a quantum computer to simultaneously explore multiple pathways.
What is Entanglement?
When quantum particles synchronize their measurements, it's termed as entanglement. In this state, measurements taken from one qubit can inform conclusions about other units. Entanglement facilitates quantum computers in tackling more extensive problems and processing larger volumes of data and information.
What is Quantum interference?
While qubits undergo superposition, they can also inherently encounter quantum interference. This interference represents the likelihood of qubits collapsing into particular states. Given the potential for interference, quantum computers strive to minimize it to guarantee precise outcomes.
How do Quantum computers operate?
Qubits and computational algorithms are employed in their operation
Quantum computers revolutionize information processing compared to classical computers. While traditional computers rely on binary bits, quantum computers utilize qubits for transmission. The qubit's capability to maintain superposition lies at the core of quantum computing's potential for exponentially heightened computational prowess.
In quantum computing, various algorithms are employed for measurements and observations. These algorithms are provided by users, prompting the computer to generate a multidimensional space housing patterns and individual data points. For instance, when tackling a protein folding conundrum to ascertain the optimal energy usage, the quantum computer assesses various fold combinations, with this combination representing the solution to the problem.
They depend on specialized computer infrastructure tailored for Quantum processing
A genuine quantum computer is primarily composed of three components. The initial component encompasses a conventional computer and infrastructure responsible for executing programming and dispatching instructions to the qubits. The second component involves a mechanism for transmitting signals from the computer to the qubits. Lastly, there must be a storage unit for the qubits. This storage unit is essential for stabilizing the qubits, and it must meet specific criteria or requirements. These requirements may include maintaining near-zero temperatures or housing within a vacuum chamber.
They necessitate physical isolation and cooling mechanisms
Qubits, as it appears, demand more maintenance than even the most volatile rock stars. Various simple actions or factors can trigger error-prone qubits to undergo decoherence, resulting in the loss of their quantum state. Activities such as measuring qubits and executing operations can lead to a crash in a quantum computer. Essentially, any utilization of the system can induce this effect. Even minor vibrations and fluctuations in temperature can prompt qubits to undergo decoherence.
To mitigate these issues, quantum computers are kept in isolation. Those utilizing superconducting circuits, the prevalent method favored by industry leaders like Google and IBM, must be maintained at temperatures close to absolute zero, approximately -460 degrees Fahrenheit.
What problems can Quantum computing address?
Can quantum computing enhance problem-solving through the utilization of quantum computers to execute quantum-inspired algorithms? Such enhancements hold potential applications across scientific and industrial domains, where considerations such as cost, quality, and production time are paramount. The advent of quantum computing could usher in novel approaches to managing air traffic control, package deliveries, energy storage, and beyond.
Climate and energy optimization problems
There's a belief that quantum computers could aid in mitigating climate change by enhancing carbon capture. Jeremy O’Brien, CEO of PsiQuantum in Palo Alto, suggested that quantum simulation of larger molecules, if successful, might facilitate the development of a catalyst capable of directly removing carbon dioxide from the atmosphere.
Molecular Modeling and Simulations
A significant advancement in quantum computing occurred in 2017, when IBM researchers successfully modeled beryllium hydride, marking the largest molecule simulated on a quantum computer at that time. Another notable milestone was achieved in 2019 by IonQ researchers, who utilized quantum computing to simulate a larger molecule, a water molecule.
Artificial intelligence breakthroughs
There is optimism that the advancement of large-scale quantum computers will expedite the progress of artificial intelligence technologies, and conversely, although opinions among experts vary on this matter. "The reason for the controversy is that things need to be reconfigured in a quantum realm," explained Rebecca Krauthamer, CEO of Quantum Thought, a quantum computing consultancy. "We cannot simply transfer AI algorithms from classical computers to quantum computers because the fundamental rules are entirely different."
Challenges in Quantum computing
Quantum noise interruptions
Presently, we find ourselves in the NISQ era, denoting Noisy, Intermediate-Scale Quantum computing. Quantum noise encompasses disruptions that impact the state of qubits, jeopardizing superposition, entanglement, and the overall precision of quantum systems. This noise stems from various factors such as temperature fluctuations, electromagnetic interference, or mechanical disturbances, rendering the maintenance of quantum computers in a proper quantum state exceptionally challenging. Consequently, NISQ computers lack the reliability required for making decisions of significant commercial importance, thus they are predominantly utilized for research and educational purposes.
Scaling and implementing Quantum technology pose significant challenges
While quantum computing holds promise for tackling intricate problems, its operational efficacy and the requisite number of qubits for such tasks remain demanding, impeding its scalability. According to Jonathan Carter, a scientist at Lawrence Berkeley National Laboratory, the challenge regarding qubits is twofold. Firstly, individual physical qubits must exhibit improved fidelity, achievable through enhanced engineering, optimal circuit layout discovery, and component combination optimization. Secondly, these qubits need to be organized to form logical qubits.
Estimations suggest that hundreds to tens of thousands of physical qubits are necessary to create one fault-tolerant qubit. Carter believes that current technologies lack the capability to scale to such levels. For instance, the computation of "the chemical properties of a novel substance" would demand millions of qubits alone, as noted by theoretical physicist Sabine Hossenfelder.
Despite these hurdles, the conceptual foundation exists to overcome them. "A quantum computer inherently understands quantum mechanics, allowing for the programming of another quantum system's functionality," explained Donohue.
Moreover, the challenges faced by quantum computing extend beyond hardware limitations. As Greg Kuperberg, a mathematician at the University of California, Davis, emphasizes, the "magic" of quantum computing lies in algorithmic advancements rather than raw speed. "If a new algorithm is devised for a fitting problem, the speed can be exponentially faster," he underscored, using "exponential" in its literal sense.
Standards for Quantum computing are currently under development
Another unresolved query pertains to the adoption of a standard method for quantum computing. Although superconducting technology has shown significant progress to date, researchers are investigating alternative approaches such as trapped ions, quantum annealing, or topological qubits. According to Donohue, the focus isn't necessarily on determining which technology is superior, but rather on identifying the most suitable approach for various applications. For example, superconducting chips align well with the magnetic field technology utilized in neuroimaging.
Insufficient expertise in Quantum computing
A significant obstacle facing quantum computing, as highlighted by Krauthamer, is the widespread scarcity of expertise. "There's simply insufficient personnel engaged at the software or algorithmic levels within the field," she noted. Tech entrepreneur Jack Hidarity's team attempted to quantify the workforce in quantum computing and discovered only around 800 to 850 individuals, as per Krauthamer. "This shortage of skilled professionals is a more pressing concern to address, even more so than hardware," she emphasized, "since it's the people who will drive innovation forward."
Why is Quantum computing significant?
Quantum computers can assess outcomes from classical computers
The practicality of research and development for quantum computers is evident, albeit on a notably limited scale. Donohue references the molecular modeling of lithium hydrogen as an example. While this molecule's size permits simulation using a supercomputer, quantum simulation offers a valuable opportunity to validate results obtained from classical-computer simulations.
These endeavors typically address relatively small-scale problems that can still be verified using classical simulation techniques. However, as Donohue points out, this progress lays the groundwork for tackling more challenging problems that would otherwise require costly large-scale particle physics experiments.
Quantum computing has the potential to revolutionize cryptography
Quantum computers have the potential to disrupt our current systems, with the RSA cryptosystem serving as the foundation for various privacy and communication protocols, including email and online transactions. Present standards rely on the assumption that no entity possesses the computational capacity to exhaustively decrypt encrypted data. However, a mature quantum computer could potentially attempt every decryption option within a short timeframe.
It's crucial to emphasize that quantum computers have not yet attained this level of maturity, nor will they for the foreseeable future. However, once a large, stable quantum device is developed, its unparalleled ability to factor large numbers could render the RSA cryptosystem obsolete. Fortunately, this technology remains distant on the horizon, and experts are actively addressing the challenge.
Mike Brown, CTO and co-founder of ISARA Corporation, a company specializing in quantum-focused cryptography, advises against panic. He reassures anxious prospective clients that the threat is not immediate, and suggests a planning horizon of 2026 to 2030 for system readiness, based on insights from the academic and industry communities.
ISARA's cryptographers are among several groups participating in the Post-Quantum Cryptography Standardization project, a competition aimed at developing encryption schemes resistant to attacks from large-scale quantum computers. Initiated by the National Institute of Standards and Technology in 2016, the project is preparing for its third round.
The complexity and stability required for a quantum computer to execute the RSA attack are exceedingly high. Nevertheless, debates persist within the quantum computing community, particularly regarding scalability timelines.
Shaping Quantum computing's tomorrow
Quantum computers do exist and are currently in use. However, they are not yet fulfilling grandiose promises such as solving climate change or revolutionizing financial forecasting. While quantum computing may eventually have commercial applications for these challenges, such advancements are still on the horizon.
"The technology isn't quite advanced enough to offer a computational advantage over other methods of computation at the moment," said Donohue. "Most commercial interest is focused on the long term. Companies are familiarizing themselves with the technology so that they are prepared when it does become more advanced — although the timeline for this advancement is fiercely debated."
Despite the fact that widespread commercial use of quantum computing is still some way off, enthusiasts can currently experiment with the technology. Small-scale quantum processors can be accessed via the cloud through platforms such as IBM's Q Experience and its open-source software Quiskit. Similarly, Microsoft and Amazon offer their own platforms — Azure Quantum and Amazon Braket, respectively. Additionally, there are resources available for learning about quantum algorithms, such as the Quantum Algorithm Zoo, which features over 60 algorithms compiled by Microsoft quantum researcher Stephen Jordan. "This accessibility is one of the exciting aspects of quantum computing today," noted Krauthamer. "We can all engage with and explore it."